Over the course of three months, Professor Michael Levine’s lab at the Department of Molecular Biology and the Lewis-Sigler Institute for Integrative Genomics have published two papers elucidating the mechanisms of genome organization in fruit flies (Drosophila melanogaster).
In a study published in the Feb. 4 issue of Science, Levine’s team discovered that two independent classes of regulatory sequences, tethering elements and insulators, organize the Drosophila genome.
Genes are basic units of heredity. Enhancers regulate gene expression (like on-and-off switches), and are scattered throughout regions of the DNA. For effective gene expression to occur, distant enhancers must come into physical proximity with their corresponding gene.
The question of how communication happens over long distances in the genome has puzzled scientists for a long time. Researchers currently believe that the DNA assumes a loop conformation to bring the necessary elements for gene expression together. However, how these loops form and what mechanism governs enhancer specificity remain unclear.
“For many years, my lab has worked on the basic logic of how enhancers work, and sometimes they map very far from the genes they regulate,” said Michael Levine, Princeton’s Anthony B. Evnin ’62 Professor in Genomics, professor in the Department of Molecular Biology, and director of the Lewis-Sigler Institute for Integrative Genomics. “And that made us think that maybe genome organization is important for allowing remote enhancers to switch their target genes on; maybe they need a little help,” he added.
Previous studies have highlighted the presence of two types of structures in the genome involved with organization.
The first class of genome organizing elements, insulators, act as boundaries, preventing physical interactions between certain DNA sequences. Insulators were first discovered by Rebecca Kellum as a graduate student in Princeton professor Paul Schedl’s lab. In a 1991 paper published in Cell, Kellum and Schedl described insulators as sequences that “segregate the chromosome into units of independent gene activity.”
The importance of insulators in mammalian development was first documented by University President Emerita Shirley Tilghman.
The second class of organizational structures are tethering elements, which connect the regions of the DNA physically far away from each other by forming loops. For a long time, it was unclear to scientists what specific features of the genome drive the formation of these loops and whether they are unique regulatory sequences themselves or belong to the family of enhancers.
It was Levine’s lab that first coined the term “tethering elements” in the early 2000’s at the University of California, Berkeley.
“We actually had the very first evidence for something like tethering elements in the late 90s, but we couldn’t do much back then,” Levine said. “And then in 2002 and 2003 my lab, then at Berkeley, published two papers in [the Proceedings of the National Academy of Sciences], actually using the term tethering element for the first time.”
Since then, the structure of insulators and tethering elements has been described to some extent, but it has continued to puzzle scientists as to their exact role in genomic regulation.
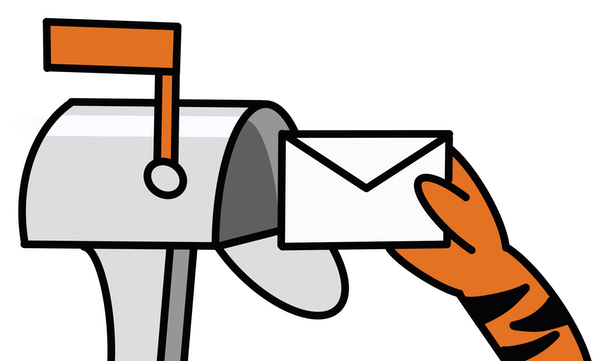
The team’s goal was to unravel the function of these regulatory elements during development.
“We tried to understand how the three dimensional organization of the genome impacts the timing and the dynamics of gene regulation in the context of development,” said the lead author of the Science paper Philippe Batut, an associate research scholar at the Lewis-Sigler Institute for Integrative Genomics. “This is truly unique. It has never been done before.”
The team’s findings demonstrated that insulators and tethering elements play diametrically opposing functions in the genome. Tethering elements mediate long-range communication between enhancers and their corresponding gene, while also fostering fast activation kinetics. Meanwhile, insulators act as gatekeepers, preventing physical interactions between enhancers and neighboring non-corresponding genetic sequences.
The collaborative function of these two regulatory elements demonstrates the critical role that the three-dimensional organization of DNA plays in the regulation of gene expression.
“It seems like [tethering elements and insulators] really form the infrastructure upon which regulation can happen at the right time and place,” Batut said.
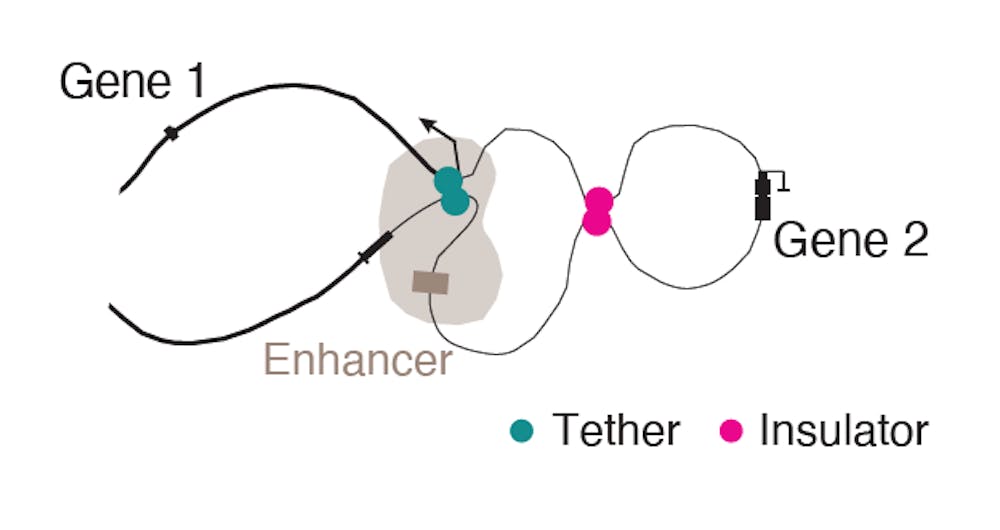
The paper mainly focuses on the contribution of tethering elements in the long range communication of a Hox gene called Scr. Hox genes are important for embryonic patterning in flies and humans.
The team chose Drosophila as their model organism since its genome is relatively more responsive to genetic manipulation. The Drosophila embryo is also transparent and grows outside the mother’s body, enabling the researchers to observe its embryogenesis.
Levine’s team’s work could have critical implications in treating a host of human diseases, from psychiatric disorders to cancer and diabetes. Batut pointed out that “a lot of mutations [that cause disease] are actually not in the protein coding sequence of the gene itself but … in the switches.”
In other words, even though there are no problems with the “light bulb” (the gene itself), a failure of the switches to function normally can disrupt the entire system.
Other exciting applications lie in the field of synthetic biology. “Having one additional type of regulatory sequence that can help foster gene regulation in a precise and more effective manner is decidedly an exciting prospect in building synthetic systems,” Batut said.
At the same time that Batut was studying the Scr gene, João Raimundo and Michal Levo, both Associate Research Scholars at the Lewis-Sigler Institute for Integrative Genomics, were looking at gene-to-gene interactions.
On May 4, Levine’s lab published a second paper in Nature, titled “Transcriptional coupling of distant regulatory genes in living embryos,” demonstrating how tethering elements coordinate gene expression during the development of the early Drosophila embryo.
In order for development to progress correctly, different genes need to be turned on or off in different places at different times — similar to a switchboard. In most organisms, the set of genes involved in development constitute a group of highly similar genes that play interconnected roles in embryonic development. This phenomenon is referred to as gene duplication.
“It’s been known for almost 100 years by now that a major force in evolution is gene duplication. You start with an ancestral gene, and that duplicates, and now you have two genes that can diverge, and give you protein products with slightly different functions,” Levine said. “That's what my lab was working on, looking in depth at two different examples of long range gene duplication events,” he added.
Although duplicates of a gene are typically found on the same region of the chromosome, the linear distances between them is usually large, with distances of up to 250 kilobases.
The surprising finding of the team is that even when the duplicated genes are located far apart, they seem to show highly coordinated gene activity. This happens as a result of a specific region of the two genes — called the promoter region — physically associating with each other. Like enhancers, promoters play a key role in the activation of gene expression; however, they are located near their corresponding genes.
The element bringing the two genes together is a familiar one: tethering elements.
This paper connects to the Science paper in the sense that while the former deals with the role of tethering elements in long-range enhancer-promoter interactions, the Nature paper shows that tethering elements also mediate long-range promoter-promoter associations.
“It’s fascinating that if you stretch the DNA in one cell, you can circle the Earth numerous times, but then you’re shoved within this container that is really small. So you have to push all of it together,” Levo said. “And that’s something that people realized a long time ago, but they didn't know whether cramming [the DNA] into that tiny space actually has some functional benefits.”
“I think what our study did is kind of help clarify this phenomenon. Because it turns out that when you shove that entire DNA into this compact container, there are genes that now find themselves in contact. Suddenly, these genes can create a specific interaction, one on one, where they talk to one another, and one switch can now coordinate their activity,” she added.
For this work, the team started by looking at genome topology, which focuses on how different points of the DNA interact in a three-dimensional manner.
“To look at contact frequencies between two molecules of the DNA, we used an assay called the Micro-C assay,” Raimundo said. “By the end, basically, we have this beautiful map of the genome.”
Among the focal contracts, points where two parts of the DNA come in contact with each other, the researchers noticed that a high percentage corresponds to promoter-promoter connectivity.
Raimundo’s “beautiful map” enabled the researchers to identify 56 instances of long-range promoter-promoter interaction during the Drosophila development over the span of one hour. The team ultimately discovered that by allowing multiple genes to be regulated using a shared enhancer, tethering elements allow the co-expression of related genes during development.
“The coolest thing I think is that not only can you see [the genes] coming together, but you can see them being turned on together,” Raimundo said. “We see that these genes … are kind of dancing together in the genome, being turned on and off, on and off, a little bit like an opera.”
Levine reflected on the significance of these two projects being done at Princeton.
“You know, Princeton is a fabulous place to study genome organization and gene function. We have tremendous depth in this area of research,” Levine said. “I'm very excited that this research on tethering elements came together here. I just feel like it's kind of cool that we're contributing this research to this landscape of excellence in this area.”
Mahya Fazel-Zarandi is a staff writer for the ‘Prince.’ She can be reached by email at mahyaf@princeton.edu or on Twitter @MahyaFazel.