Adam Burrows is a professor of astrophysics at the University and has served on the Board of Trustees of the Aspen Center for Physics. In the past, he was the chair of the Board on Physics and Astronomy of the National Research Council and has worked on a number of committees for NASA.
Recently, Burrows was awarded the 2020 Viktor Ambartsumian International Science Prize “for his seminal and pioneering contributions to the theories of brown dwarfs and exoplanets and for his leadership role in educating a generation of scientists at the frontiers of brown dwarf and exoplanet research,” according to the Prize Committee’s press release. He sat down with The Daily Princetonian for a virtual interview, touching on his work on brown dwarfs, his career path, and the importance of teaching to excel in research.
The Daily Princetonian: I’d like to start by saying congratulations for winning this prestigious prize. I understand that you won for your research on brown dwarfs and exoplanets. Could you walk me through what that work entails?
Adam Burrows: A number of decades ago, it was interesting, theoretically, to look at objects that were significantly smaller than regular stars. As you go down in mass, from one solar mass to half a solar mass, to one-third, etc., the luminosities go down significantly and the temperatures get lower and lower at the surface. When you get down to about one-twelfth of a solar mass, you get to the point where you can't derive enough thermonuclear burning in the star to balance the losses from the surface. So below that mass, you have what are called brown dwarfs. The brown dwarfs will have a little bit of thermonuclear life, but they won't be able to compensate for the losses from the surface. They're like dying embers plucked from a fire.
What I did with collaborators over the years was to calculate what these things would look like... Over the last many years, people have developed the technology necessary to characterize brown dwarfs — we have discovered a few thousand of them — but what we did early on was try to provide the theoretical context for understanding these objects. And that’s what is being recognized.
You can also ask the question, “What if you have an object that is much less massive than this transition mass, which, as I said, is one-twelfth of the solar mass, or around 70 Jupiter masses?” If you go down to 60 or 40 masses, you have brown dwarfs, but if you extend it down to five, four, three, or just the mass of Jupiter, you’re starting to talk about exoplanets. So, at the same time, we started putting together a theory about these objects, which is an extension of the work on brown dwarfs that straddles the realm between brown dwarfs and the planets we know in our solar system...
At around the same time, the first unimpeachable brown dwarf was discovered. [Michel] Mayor and [Didier] Queloz discovered 51 Pegasi b, the first exoplanet around a solar-like star, and this discovery garnered the Nobel Prize in Physics for them last year. At that time, we were the only theorists working on this general subject, and we taught a generation of theorists and observers about these objects. Collectively, the giant planet and brown dwarf work we did is the origin of this prize and the kudos that I quite gratifyingly received.
DP: What direction do you wish to take this work in the future?
AB: Important in the near future is what we can learn using the James Webb Space Telescope because it will be exquisitely sensitive to brown dwarf and exoplanet observation. There will also be another space-based satellite — Ariel — that the Europeans are going to launch towards the end of this coming decade. What we’re going to be able to understand at the lower temperatures of brown dwarf and exoplanet surfaces is cool molecular atmospheres similar to those of the local planets with which we are familiar...
I used to be the director of the Planets and Life certificate program at Princeton, which is astrobiology, and part of the subject is the connection with the origin of life. There’s a lot of study to try to understand the origin of life on Earth — there was just the launch of the Perseverance probe to Mars, part of the tradition of Mars probes to search for signs of past life — but it would also be nice to have other targets outside of the solar system, where we may be able to discern signatures of life. That’s a goal, and it’s been a goal of a good fraction of astronomy and planetary science for a long time.
DP: I know your many other research interests include impressive topics such as nuclear astrophysics and supernova theory, so what’s another particularly memorable research experience or project from your career, and could you tell me a bit about it?
AB: I’m still working a lot on supernova theory, and what we’re trying to do is to understand the mechanism of explosion. Supernovae are important agencies of change in the universe. They’re the source of many of the heavy elements in nature. The iron in your hemoglobin, the calcium in your bones, the oxygen you breathe, and the fluorine in your toothpaste come from the massive stars that explode in supernovae.
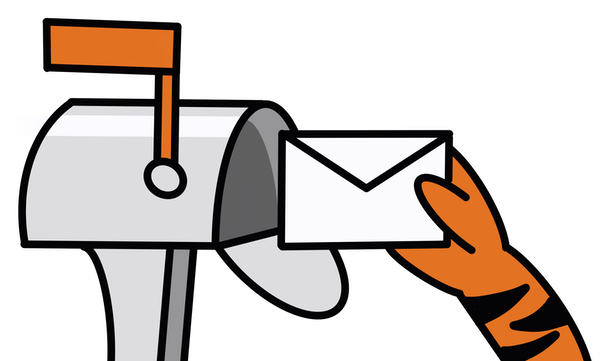
The galaxy is constantly enriched by these heavy elements, and the solar system and the Sun are actually the products of this progressive enrichment. But the mechanism of these explosions has been shrouded in mystery because they happen in the deep interior of a star, which we don’t have access to directly. By dint of nuclear physics, particle physics, and large supercomputers, we’ve recently been able to simulate in some detail the internal dynamics of this object that gives us a supernova.
DP: How did you get your start in astrophysics? What drew you to the field?
AB: I was interested in how things work and in physics, and what I liked about physics was its broad applicability. But I didn’t want to major in just one aspect of physics. I wanted to range broadly, and you can do that in astrophysics. You learn a little bit about everything and bring it together — it’s at the interface of many of the disciplines in physics — and in the process, you learn how nature works, because it doesn't silo these disciplines, but combines them effortlessly.
DP: How do you think we should approach the search for life in space, with issues like both forward and backward contamination to consider? Also, some scientists suggest first coming up with an accurate definition of life before continuing our search in space. What are your thoughts on that?
AB: It’s a much discussed topic: You’re talking about planetary protection and contamination, both backwards and forwards. People worry about that, but I’m not as worried — I think people have been pretty careful. But over time, with commercial space initiatives and with the multitude of countries that are getting involved, the solar system is going to be contaminated. So we better hurry up if we’re trying to understand the origin of life in the solar system.
Having said that, you also want to have a protocol for understanding what the atmosphere of a life-bearing planet looks like — the so-called biosignature. There’s a lot of caveats there — do you really have a general theory about what life’s products will be? Do you really have a general theory of the evolution of life in many different contexts? And there could be very many contexts that could give rise to self-replicating organic lifeforms that satisfy Darwinian evolution. It’s something that requires thought in all directions, and I certainly wouldn’t want to stop things just to contemplate how best to proceed. The most important thing is to start getting data, both in the solar system and beyond.
DP: How was your experience in working with NASA, with roles such as co-chair of the organization’s Universe Subcommittee?
AB: You learn how the sausage is made, which is probably the most important thing, but you also get an appreciation for how hard a lot of these things are, and how good and professional of an organization NASA is. No organization is perfect, but it’s been quite successful. It’s well-configured to answer many of the questions that many of us have about the universe...
DP: How does teaching inform your research, and vice versa?
AB: You really need to be connected to students, or you don’t get the energy that they provide. You need to collaborate with students, not only because that gives you a means to get work done, but it also sparks ideas. It’s only in the academic environment that you’re challenged by new results coming in all the time, and the ivory tower isn’t the best place to do real science — mathematics, perhaps. You need to be engaged, and you’re best engaged in an academic context, which involves students. And your involvement with students and their involvement with you is central to real progress in science.