In 1936, the work of Alan Turing GS ’38 laid the groundwork for the birth of the first modern computer. Today, University researchers led by physics professor Jason Petta are inching towards what could be another revolution in the field: the quantum computer.
Petta’s team has developed a procedure to measure the spin state — or rotational properties — of electron pairs, potentially allowing quantum information to pass through a computer rapidly and reliably, much as electrical information already flows in classical computers today. The finding was published last month in the journal Nature.
While measuring the electrons’ spin states, the researchers spray microwaves on a nanowire, a thin rod that traps the electrons. Based on the changes in this microwave field as it interacts with the electron pair, the research team can determine whether the spins of the electrons are the same, known as parallel, or different, known as antiparallel.
Petta’s method relies upon a law known as the Pauli Exclusion Principle, which states that no two electrons with the same spin can occupy the same space.
“Imagine a bar magnet with a north pole and a south pole,” Petta said. “Each electron spin is kind of like a bar magnet, but on a much smaller scale. If the spin states are parallel, the electrons can’t occupy the same space; when they’re antiparallel, they can. That’s what we can measure with microwaves.”
A quantum mechanical principle known as superposition underpins the key architectural difference between quantum computers and their classical counterparts. According to superposition, small enough systems — like individual electrons — can exist in several states at the same time.
To store information and perform complex calculations, today’s computers use “bits” which can take values of only 0 and 1. Quantum computers use “qubits,” electron pairs that can take values of 0, 1 or any value in between.
This fundamental difference might allow quantum computers to produce solutions to problems a classical computer would never be able to solve, according to electrical engineering professor Andrew Houck, who was a co-author with Petta on the study.
“Quantum computers don’t outperform classical computers in every scenario, but there are certain problems where quantum computers are fantastically powerful,” Houck said. “Quantum computers don’t use quantum mechanics to perform each step of a calculation any faster, but in some situations, quantum computers require fewer total steps to produce a solution."
One of the most direct applications of the quantum computer is in the field of digital security.
A common system of encryption relies on the premise that extremely large numbers are impossible to factor in a reasonable amount of time. For instance, a 200-digit number takes several decades to separate into its prime factors using a standard desktop computer. A quantum computer, however, might be able to complete this same task in a matter of hours.
This would make it easier to crack security codes on computer systems.
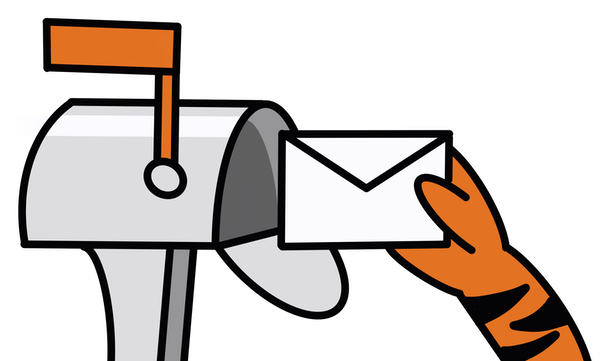
The next step for scientists working on quantum computing is to construct systems consisting of more than a single qubit. In order for quantum computers to be of practical use, experts say they will need to consist of 100 qubits.
Petta acknowledged that getting up to this scale might present complications, but he said he was optimistic that his team’s technique could handle a greater number of electrons.
“You can’t do much that’s exciting with a quantum bit; we want quantum bits,” Petta said. “At the end of the day, there’s just a lot of real estate on this chip to put on a lot more semiconductor devices.”